The nuclear spin polarization
The nuclear Zeeman effect is a very weak interaction. Thus, it effects also very small energy splittings of the spin states within technically achievable magnetic fields. This situation has several consequences. On the one hand, the long natural lifetimes of the excited spin states and the resulting long decoherence times cause extremely narrow homogeneous line widths. On the other hand, the small Boltzmann factor leads to extremely small polarization of the spin transition. As the population difference between the energetic ground and the excited state, this polarization directly limits the reachable NMR signal intensity of a system in thermal equilibrium. Together with the slow return of the spin system into equilibrium after a radio frequency pulse (relaxation), this results in large experimental time requirements for recording NMR spectra with sufficient signal-to-noise ratio.
DNP: Transfer of electron spin polarization to nuclei
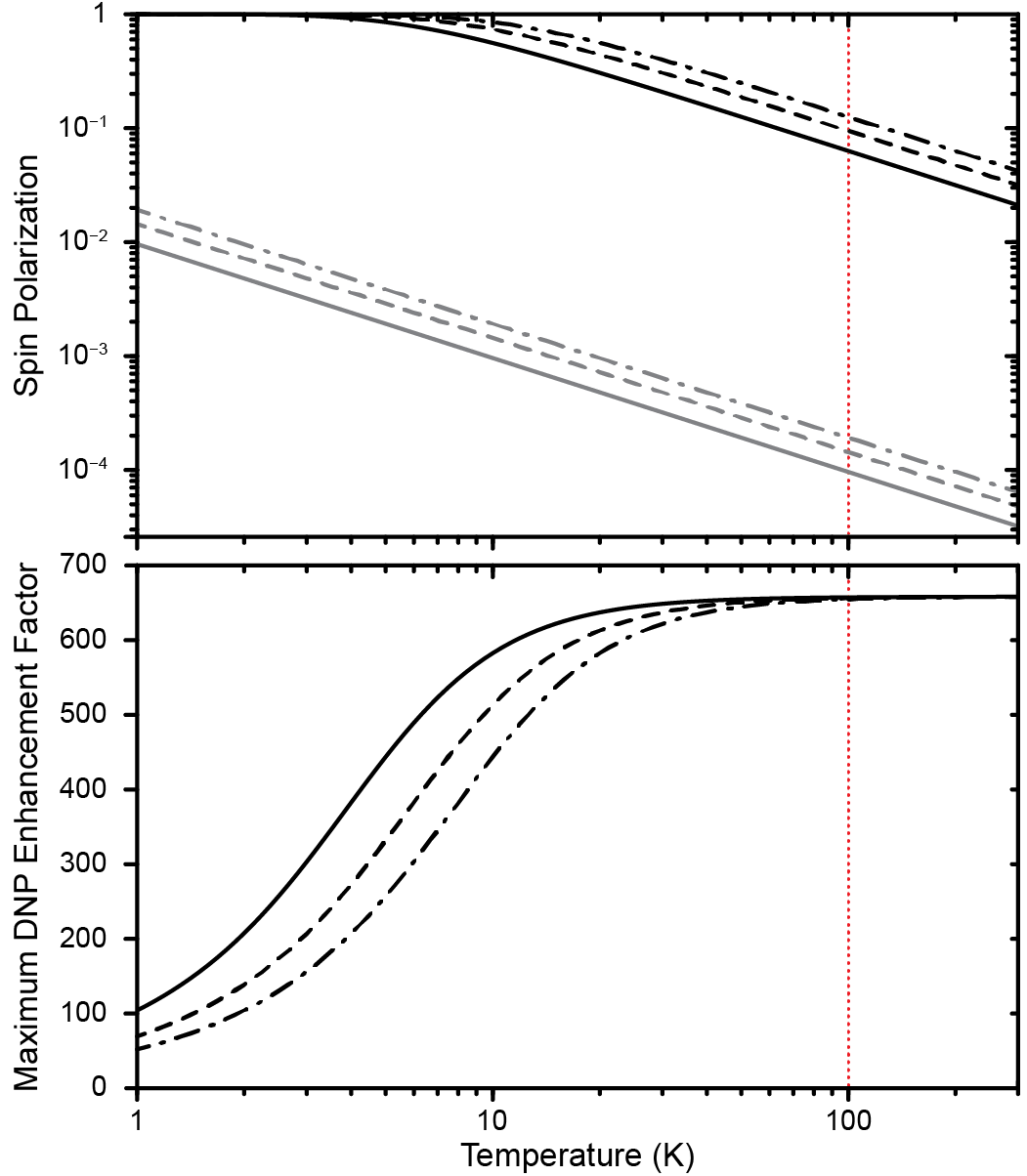
The thermal spin polarization of unpaired electrons is about three orders of magnitude larger as compared to nuclear spins. Through dynamic nuclear polarization (DNP) this large polarization may be transferred from electrons to nuclei and the NMR sensitivity can be increased. For 1H, the maximum DNP enhancement factor is about 660, for other nuclear spins this factor is larger according to their gyromagnetic ratio. However, losses due to relaxation and inefficiencies of transfer mechanisms result in typical enhancement factors of 20-200. Nevertheless, this factor effects an acceleration of NMR experiments by a factor of 400-40,000!
DNP mechanisms
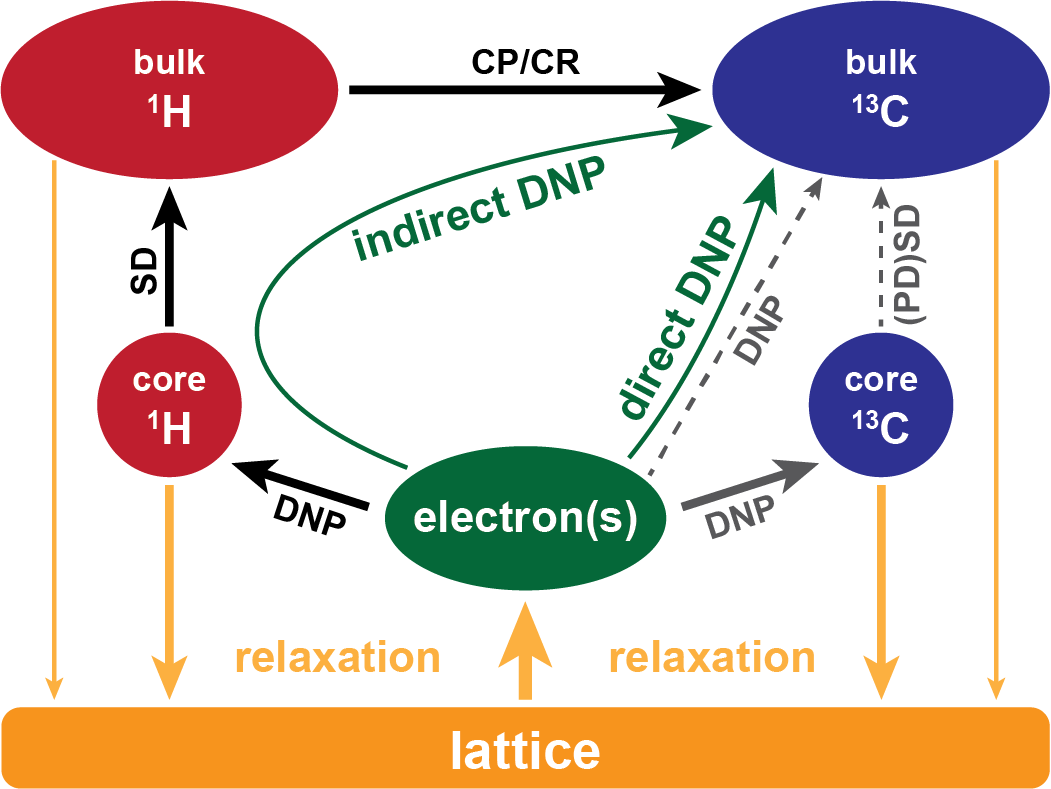
In solids there a several DNP mechanisms. Of those, the solid effect (SE) and cross effect (CE) are the two most relevant.
- The solid effect has been the first known DNP mechanism to occur in electrically non-conductive (dielectric) solids. It is based on the direct excitation of zero and double quantum coherences of an electron and a nuclear spin which are nominally forbidden due to quantum mechanics. Therefore, strong microwave fields are required for their excitation and the efficiency of SE is consequently limited, particularly at high magnetic fields. Nevertheless, the SE is an important DNP mechanism due to its simplicity regarding the required minimal spin system.
- The cross effect is very efficient and the most commonly evoked DNP mechanism. Here, uniquely tailored polarizing agents are utilized which contain two unpaired electrons (weakly coupled biradicals). If the EPR spectrum of one of the radical moieties is saturated by microwave irradiation, the polarization of the second electron may be transferred onto a nucleus due to magnetic coupling between the electron spins. This is particularly efficient during MAS.
Polarizing agents
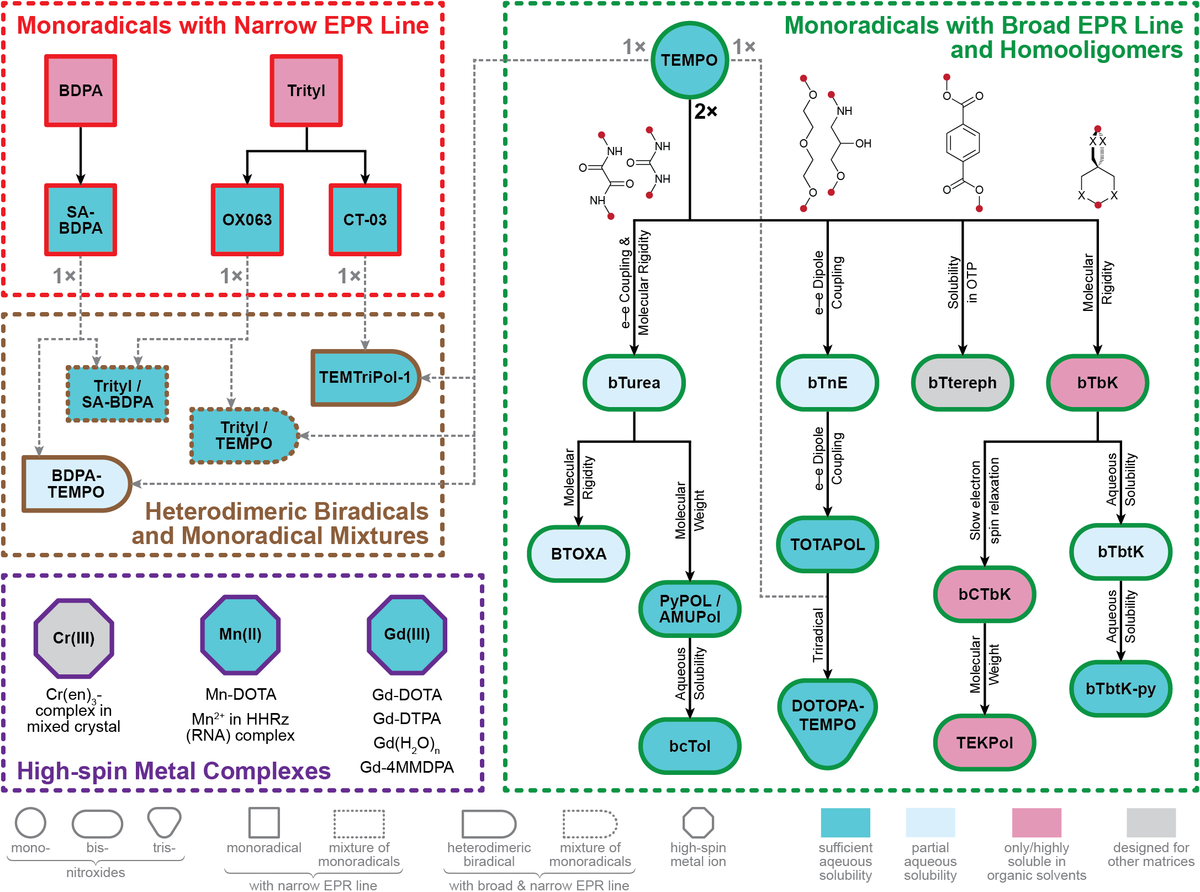
The spin of unpaired electrons serves as the source of the large polarization which is transferred to nuclei during DNP. However, unpaired electrons in molecules are only chemically stable under certain conditions. These may be provided, for example, by sterical hindrance or mesomeric stabilization within persistent radicals, or by the intrinsically higher stability of the deeper lying open electronic shells of transitions metal ions or lanthanoids.
For SE; simple radicals such as trityl (triarylmethyl) or BDPA (1,3-bisdiphenylen-2-phenylallyl) are utilized. Alternatively, metal ion complexes of Gd3+ or Mn2+ may be used. For CE, nitroxides are tethered to dimers, most commonly on the basis of TEMPO [(2,2,6,6-tetramethylpiperidin-1-yl)oxyl], or a linked with trityl or BDPA to heterodimeric biradicals. Solubility in different environments is achieved by suitable derivatization.
MAS-DNP hardware
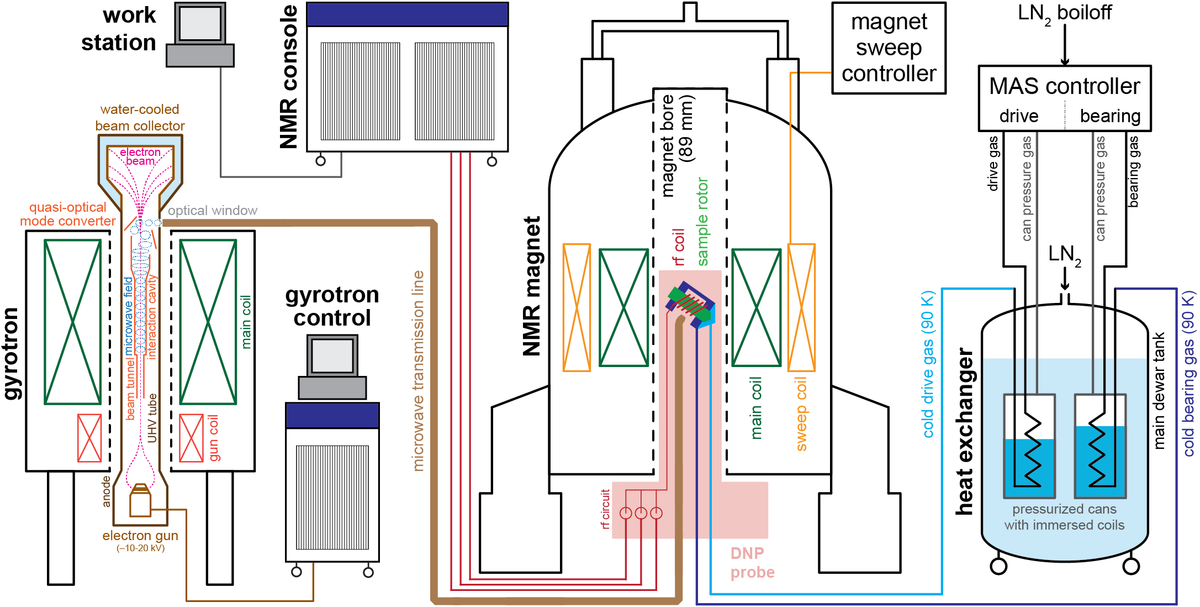
Besides the central MAS-NMR spectrometer, DNP requires additional components: (1) the microwave source, (2) a DNP probe, and (3) a cryo-MAS heat exchanger.
- A gyrotron is most often used as the microwave source for DNP. In this cyclotron electron beam MASER device, linearly accelerated electrons are injected into a magnetic field whereupon radiation with frequencies between approx. 100 and 1000 GHz may be generated at a power of up to ~100 W. The capability of generating that large microwave power at high frequencies is unique and has revolutionized MAS DNP during the 1990 decade. Once generated, the microwave beam is led to the DNP probe via an overmoded circular waveguide.
- The DNP probe is based upon a conventional MAS NMR probe but features several necessary modifications. First, a waveguide allows the irradiation of the sample during MAS with microwaves and therefore the induction of a suitable DNP mechanism. Second, thermal insulation and the capability of rapid rotor eject and insert without removal of the probe is required because DNP is typically performed at cryogenic temperatures around –173°C (100 K). The sample cooling freezes molecular motion and thus guarantees a rigid spin network which allows the spreading of enhanced nuclear magnetization via spin diffusion; additionally, slowing of spin relaxation increases the DNP efficiency.
- The cryo-MAS heat exchanger uses liquid nitrogen at a temperature of 196 °C (77 K) for cooling of gaseous nitrogen which in turn is used as MAS bearing and drive medium. This allows the lowering of the sample temperature down to –173 °C (100 K) and below.